Gaia and the Race to Detect More Gravitational Waves
Gravitational waves (GW’s) are disturbances in spacetime produced by any massive object moving asymmetrically. However, only the most massive and most relativistic objects produce large enough GW’s to be detectable. The Laser Interferometer Gravitational-Wave Observatory (LIGO) and Virgo detectors are using laser interferometry to detect tiny ripples in the fabric of spacetime. They have detected dozens of GW’s from binaries of black holes and neutron stars. An additional method of detecting GW’s is creating a pulsar timing array (PTA), where dozens of millisecond pulsars are monitored to look for the signatures of nanohertz GW’s, that is waves with much lower frequencies than those seen by LIGO and Virgo.
Another way of detecting GW’s is using astrometry, this method measures the apparent positions of stars. This method, first introduced in 1990, and these days it is getting more relevant due to the Gaia mission, which makes astrometric observations at unprecedented precision.
Table of Contents
Key Points
- Gravitational waves (GW’s) are disturbances in spacetime produced by massive objects moving asymmetrically.
- Laser Interferometer Gravitational–Wave Observatory (LIGO) and Virgo detectors use laser interferometry to detect GW’s.
- Pulsar timing arrays (PTA) use dozens of millisecond pulsars to look for the signatures of nanohertz GW’s.
- Astrometry detects the apparent positions of stars, and is used to detect GW’s. 5. Gaia mission makes astrometric observations at unprecedented precision.
- GW’s modulate spacetime and cause the apparent position of objects in the sky to change with time.
- Scientists introduced a method to compress the Gaia dataset by a factor of 1 million.
- Sensitivity of the Gaia dataset is comparable to that of pulsar timing arrays and may even compliment them at higher frequencies.
- Most sensitive regions are around the galactic poles and least sensitive regions are towards (and away from) the galactic centre.
- Gaia may assist in understanding supermassive black hole binaries in distant galaxies by detecting their GW’s.
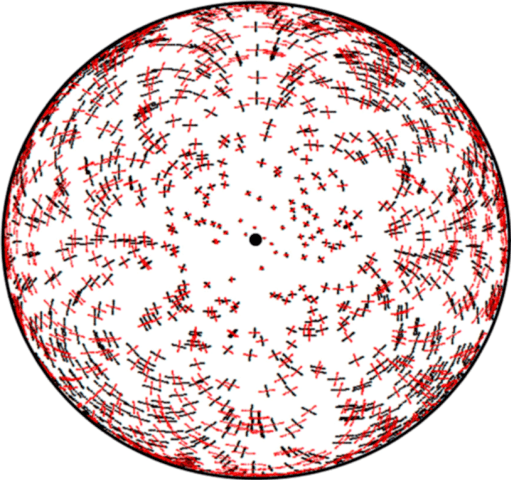
Figure 1: Astrometric response to a GW coming from the sky location marked with the black dot (centre). Black and red lines indicate the movement of stars for plus and cross-polarized GW’s, respectively. Note the astrometric effect of a GW is the strongest perpendicular to its direction of propagation. Also, the GW amplitude is unrealistically large here to make the effect visible.
Stars shaken due to passing GW’s: How can astrometry assist with GW’s?
Imagine a long time ago in a galaxy very distant, two supermassive black holes were orbiting around each other, emitting GW’s. Those GW’s are reaching us today, passing through the Milky Way! They modulate the spacetime throughout the galaxy, therefore affecting how light, (photons), propagate. Thus, a GW changes the time it takes a photon to travel between objects, which is utilized by pulsar timing arrays to detect GW’s. That is not the only effect GW’s have! They also affect the propagation of light (similar to gravitational lensing around a massive object), causing the apparent position of objects in the sky to change with time (figure 1).
Gaia measures the position of > a billion stars with astonishing precision. It turns out, this is positive and negative. It helps with sensitivity to have a large amount of data, but it makes statistical analysis of the full dataset computationally infeasible. To overcome this, scientists introduce a method to compress the dataset by a factor of 1 million, while only reducing their sensitivity by 1%. They are partitioning the sky using Voronoi cells, and averaging the data from stars in each cell, resulting in one “virtual star” for every cell.
Gaia’s Sensitivity
Assessing Gaia’s sensitivity to GW’s, researchers created simulated datasets including a GW signal with different amplitudes and frequencies. Analyzing these datasets, they were able to determine the minimum amplitude needed to detect a signal at each frequency. They found the sensitivity of the Gaia dataset is comparable to that of pulsar timing arrays, and may even compliment them at higher frequencies. They also looked into how the sky location of the source affects the sensitivity (figure 2). They discovered the least sensitive parts of the sky are ~ 30% less sensitive than the best location. Notice that the most sensitive regions are around the galactic poles, while the least sensitive regions are towards (and away from) the galactic centre. It is expected from the distribution of stars observed by Gaia, and the fact that the GW response is strongest in the direction perpendicular to the propagation direction of the GW. This being the opposite of what we see for pulsar timing arrays, where the sensitivity is the best towards the galactic centre. Meaning Gaia might complement pulsar timing arrays not just at high frequencies, but also at high galactic latitudes. This result is of great interest if we think about multi-messenger astronomy since a GW detection far from the galactic plane would be more likely to lead to a successful observation of an electromagnetic counterpart.
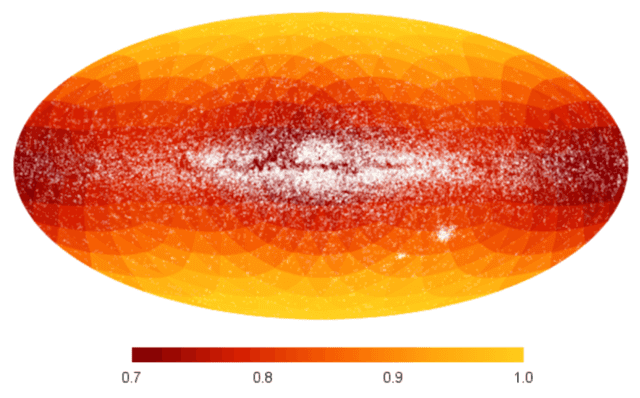
Figure 2: Sensitivity of Gaia dataset to GW’s over the sky normalized to the most sensitive sky location (redder colours indicate less sensitivity).
Gaia was designed to assist in understanding the Milky Way, however, it looks like it may also help in learning about supermassive black hole binaries in distant galaxies by detecting their GW’s. This would be an alternative use of Gaia and provides an additional reason to look forward to future Gaia data releases.
References:
Gilmore, G.
Lasenby, A.
Mihaylov, D. P.
Moore, C. J. (Department of Applied Mathematics and Theoretical Physics, Centre for Mathematical Sciences, University of Cambridge, UK).
A Nuclear Fusion Physicist and Astrophysicist.
BSc Physics & Engineering, MSc Nuclear Physics & Engineering, MSc Astrophysics, PhD Plasma Physics
Do you have numbers how much we expect each star position to change? Is this something in the range of the parallax resolution of stars, or is this more like "everything shifts by 0.001 standard deviations and we need millions of stars to be sensitive" (i.e. nano-arcseconds)?
I know it's difficult for Gaia to get relative positions of stars across large separation in the sky, and that's probably what they need here: They just have two relatively narrow fields of view 60 degrees apart, so their correlation matrix is very thin.